Next-Generation Sequencing and Single-Cell Technology: What’s the Difference?
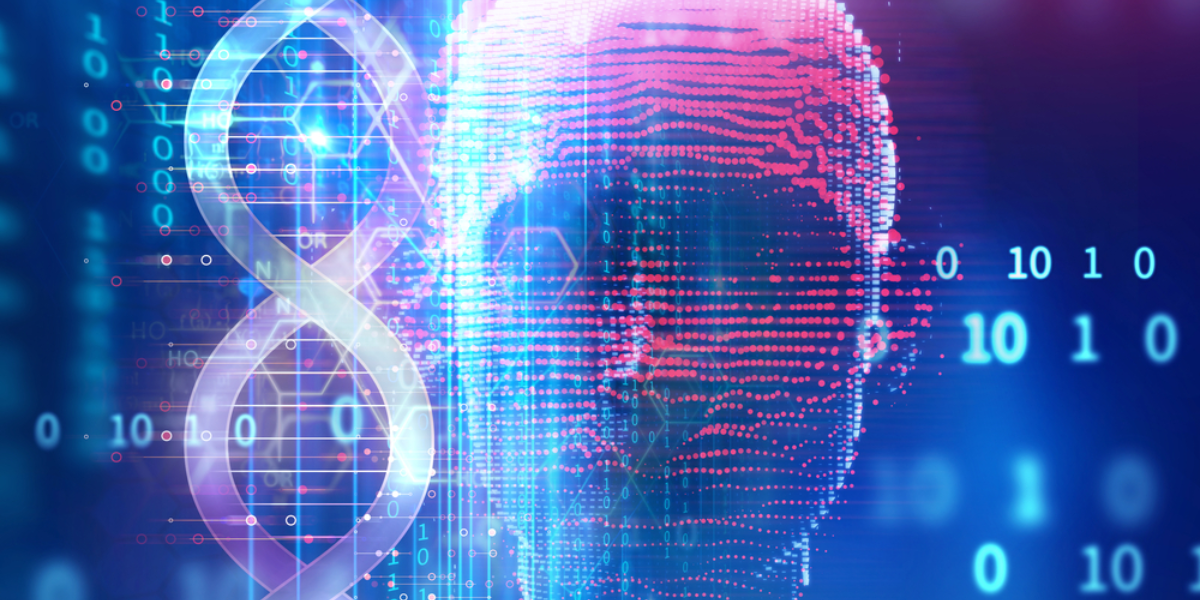
Next-generation sequencing (NGS) and single-cell technology share many overlaps, which is perhaps why they’re often confused. However, both terms sit in their own respective corners of the pentacontagon of biological understanding—that’s a 50-sided polygon, for reference.
In the same way that two books (one science fiction and one high fantasy) might be categorized next to each other in the fiction section of a library, NGS and single-cell technology contain their own world of research and understanding.
However, one easy distinction is that single-cell genomics & technology involves the whole cell, whereas next-generation sequencing only involves one piece of the cell (DNA or RNA sequencing analysis). This is, again, too simple of analysis to be accurate, but it will do as a brief introduction to the two.
Next-Generation Sequencing and Single-Cell Technology
To completely understand the difference, you need to isolate each concept. Only then will they stand out as their own pockets of research. Thus, this guide will be separated into two parts:
- Part 1: What is next generation sequencing?
- Part 2: What is single cell technology?
By the end, you should have a clear picture of both concepts, their common techniques and technologies associated, and the analytical gap between them.
What is Next-Generation Sequencing?
NGS is the overarching term that encompasses the techniques and technologies available today that allow researchers to sequence strands of DNA and RNA. This allows them to study individual sections, or genes, and identify which sections of genomes contribute to disease or biological characteristics.
How is this accomplished? And what is the specific sequencing technology and method involved?
Sequencing Step-By-Step Process
Regardless of instrumentation (NGS technologies), next-generation sequencing follows a similar workflow (NGS techniques) every time. There are four steps to the NGS workflow:
- Crafting the sequencing library – The sequencing library is created by making DNA fragments into short strands. This can be done using enzyme digestion, shearing, and PCR amplification techniques. With the help of next generation sequencing data analysis systems, these strands are individually detected and stored—like generating a barcode for each strand of DNA available.
- Amplifying the clones – This process of “building the barcodes” involves semiconductors, which translates the specific peptides (A, T, G, and C) into sequencing data that can be stored on a computer. For this, you need a lot of information. Using polymerase chain reactions, you can multiply the available DNA strands and make millions (or billions) of copies. This is known as PCR amplification.
- Reading the DNA (or RNA) strand – As the polymerized strands grow, the NGS technologies (whichever one you use), record the sequencing growth and essentially “read the DNA (or RNA) strand.”
- Analyzing the results – The raw signals coming in from the semiconductors are translated into patterned electronic data (0’s and 1’s). This is then assembled, trimmed, and formatted to fit within the sequencing library constraints. Finally, meaningful information needs to be extracted—this is where the beauty of NGS technologies comes in.
To gain more insight into the NGS workflow and processes, you need to know a bit about the actual methods involved.
Related: RNA Sequencing Analysis
Specific Next-Generation Sequencing Methods
Next-generation sequencing involves common technologies that have been used for the last 15 to 20 years. These are known as “third generation” sequencing methods—the second generation and first generation of these sequencing techniques, including Sanger sequencing, takes scientists back to the 1970s.
What are some next-generation sequencing methods?
- Whole genome sequencing – When you’re attempting to discover a novel genomic sequence, WGS is your best bet. This sequencing method creates an in-depth analysis of the entire genome and does not rely on previous sequence knowledge. While great for disease genetics analysis, anomaly detection, and aneuploidy detection, it is the slowest method and also has the highest cost of the primary sequencing efforts. High computational and data demands makes it efficient for novel discoveries, but other than that there are more targeted efforts.
If there is already genomic information available about what you’re studying, you can often use two methods that work on targeted sequences:
- Exome sequencing – If you are primarily focused on the regions of the genome associated with protein production (the exons), then you can use exome sequencing. This allows you to focus on roughly 1% of the entire genome, saving you 99% of the computing power.
- Region-specific panel sequencing – Similar to exome sequencing, the region-specific panel sequencing allows you to target individual portions of a genome. It’s the fastest of the three methods and is great for oncologists, immunologists, and researching more on known diseases. However, the targeted approach does lead to missed results if you’re not careful.
By now, you (hopefully) have a solid understanding of Next-Generation Sequencing and are ready to understand the other concept we have on our plate. Single cell technology.
What is Single-Cell Technology?
As a fundamental understanding, single cell technologies are tools within cell biology and microbiology to analyze cells and cell populations. These tools are incredibly useful when most samples that researchers have to work with come in heterogeneous populations.
Single-cell methods, such as DNA sequencing technology, are then the complementing tools to bulk analysis methods (which have long been in use).
In a 2016 issue of Nature surrounding single cell technology, they present their focus piece to describe the rising importance of single cell technology in unmasking the inherent heterogeneity of various sample tissues:
“With the increasing sophistication of microfluidics, electrophysiology measurements, high-resolution imaging, deep sequencing and mass spectrometry platforms, a more detailed picture of cellular subtype, physical location in tissue, and clonal evolution is emerging. Moreover, the exquisite sensitivity of these approaches is enabling the identification of rare cells of potential functional or pathogenic consequence.”
This offers a succinct way to describe the well of information needed to inform single cell technology.
Specific Technologies (and Techniques) Behind Single-Cell Analysis
From there, the specific technologies and methodologies will inform much about what this branch of cell biology covers.
- Single cell DNA sequencing
- Cytometry and cell sorting
- Cell cloning techniques
- Real-time PCR (polymerase chain reaction)
Single-cell DNA sequencing is considered one of the many single-cell technologies. So, while there are many differences when talking about both terms, now you can glean some insight into how they are similar.
Let’s discuss the other single-cell technologies.
Cytometry and Cell Sorting
It’s much easier to work on single-cell analysis with a homogenous population. Flow cytometers help with this.
Cytometry is a method that sorts cells based on certain characteristics in a highly efficient manner. Using properties of fluid dynamics, a cytometer is able to create a flow that allows cells within a given sample to pass through a laser one at a time (with very little couplets or error).
From there, laser optics and measuring devices are used to identify the cell, and electronics systems charge the cell either positive or negative (or remain neutral), which then isolates cells into one of three categories.
This is a primary resource in nearly all cell research labs, with the only downside being the high pressures can cause abrasion and cell death in the population.
That is, until NanoCellect created the WOLF G2 Cell Sorter. By using sorting pressures of <2 psi, it is officially 30 times gentler than industry-standard flow cytometers. Additionally, the WOLF Cell Sorter is:
- Contaminant and biohazard free thanks to its disposable cartridges
- Easy to use, with complementary software that assists beginner and experts
- Flexible and easy to squeeze into any lab (at under 2 cubic feet)
Cell Cloning Techniques
Once the cells are sorted, the process of cloning allows you to create a large homogenous sample from just a few individual cells. The techniques involved include:
- Clone generation by single-cell deposition technology
- Serial dilution method
- Cloning ring method
Real-Time PCR (Polymerase Chain Reaction)
RT-PCR allows researchers to quickly and efficiently multiply the amount of DNA strands available for analysis. This can be done on the scale of millions or billions of copies, depending on what’s needed.
The steps for RT-PCR include:
- Isolating cells
- Washing the sample with a phosphate-buffered saline solution
- Mixing the sample with lysis solution and incubated
- Releasing the RNA
- Adding stock solution to deactivate the lysis solution
Putting It All Together
You can probably see where a lot of the overlap comes into play between next-generation sequencing and single-cell technology. Both techniques are sensitive to contamination and, despite the major advances in the field, there is still room for error. Thus, single-cell and NGS technology both rely on precise, gentle handling.
This is where having the proper cell sorter comes in. NanoCellect’s WOLF Cell Sorter enables healthy, viable cells. This allows NGS, single-cell multiomics and antibody development to be done efficiently.
That’s one more thing they have in common.
Sources:
- NIH. ENCODE: Deciphering Function in the Human Genome. https://www.genome.gov/27551473/genome-advance-of-the-month-encode-deciphering-function-in-the-human-genome
- Nature. Single-cell technology. https://www.nature.com/collections/nylbrpjhlp
- NCBI. Single-cell technologies are revolutionizing the approach to rare cells. https://www.ncbi.nlm.nih.gov/pmc/articles/PMC4796591/