Protoplast isolation and enrichment using the microfluidic WOLF® Cell Sorter
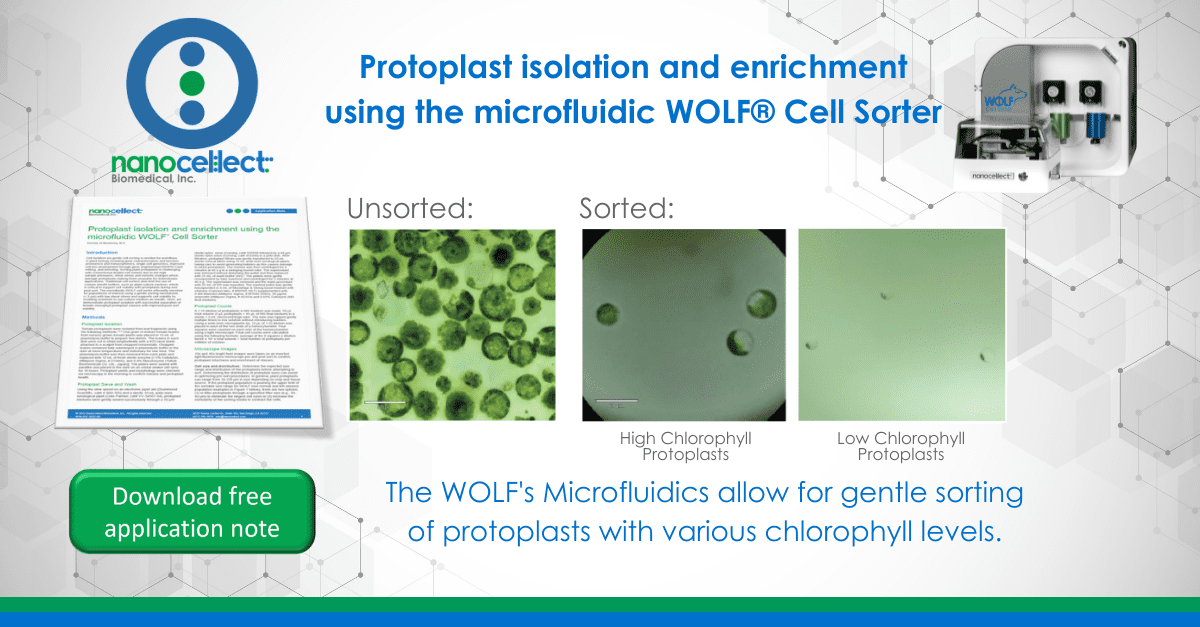
Introduction
Cell isolation via gentle cell sorting is needed for workflows in plant biology including gene characterization and function, proteomics and transcriptomics, single-cell genomics, improved cell line development through gene engineering/CRISPR-Cas9 editing, and breeding. Sorting plant protoplasts is challenging with conventional droplet cell sorters due to the high sample pressures, shear stress and osmotic changes which damage protoplasts making them unusable for downstream applications. Traditional cell sorters also limit the use of custom sheath buffers, such as plant culture medium, which is critical to support cell viability with protoplasts during and post sort. The microfluidic WOLF cell sorter efficiently enriches for populations of interest using a gentle sorting mechanism (< 2 psi) with low shear stress and supports cell viability by enabling scientists to use culture medium as sheath. Here, we demonstrate protoplast isolation with successful separation of tomato mesophyll protoplast classes with improved post-sort viability.
Method
Protoplast Isolation
Tomato protoplasts were isolated from leaf fragments using the following methods.1,2,3 One gram of mature tomato leaves from nursery grown tomato plants was placed in 12 mL of plasmolysis buffer to prepare two dishes. The leaves in each dish were cut in strips longitudinally with a #23 razor blade attached to a scalpel then chopped horizontally. Chopped leaves remained fully submerged in plasmolysis buffer in the dark at room temperature and stationary for one hour. The plasmolysis buffer was then removed from each plate and replaced with 12 mL of fresh sterile enzyme [1.5% Cellulysin, (Millipore Sigma, # 219466), and 0.4% Macerozyme (Yakult Biochemicals Co. Ltd., Japan)]. The plates were sealed with parafilm and placed in the dark on an orbital shaker (40 rpm) for 18 hours. Protoplast yields and morphology were checked via microscopy in the morning to confirm release and protoplast health.
Protoplast Sieve and Wash
Using the slow speed on an electronic pipet aid (Drummond Scientific, cat# 4-000-105) and a sterile 10 mL wide-bore serological pipet (Cole-Parmer, cat# VV-34567-54), protoplast mixtures were gently sieved successively through a 70 μm sterile nylon sieve (Corning, cat# 352350 followed by a 40 μm sterile nylon sieve (Corning, cat# 352340) in a petri dish. After filtration, protoplast filtrate was gently transferred to 50 mL sterile conical tubes using 10 mL wide bore serological pipets, taking care to avoid generating bubbles as this causes damage to intact protoplasts. The mixture was then centrifuged for 8 minutes at 46 x g in a swinging bucket rotor. The supernatant was removed without disturbing the pellet and then replaced with 25 mL of wash buffer (W5).1 The pellets were gently resuspended by tube inversion and centrifuged for 3 minutes at 46 x g. The supernatant was removed and the wash procedure with 25 mL of W5 was repeated. The washed pellet was gently resuspended in 4 mL of Murashige & Skoog basal medium with vitamins (Caisson labs, # MSP09-10LT) supplemented with 0.4M Mannitol (Millipore Sigma, # M1902-500G), 50 μg/mL ampicillin (Millipore Sigma, # A5354) and 0.05% Cellulysin (MS final medium).
Protoplast Counts
A 1:10 dilution of protoplasts in MS medium was made: 50 μL total volume (5 μL protoplasts + 45 μL of MS final medium) in a sterile 1.5 mL microcentrifuge tube. The tube was tapped gently multiple times to mix solution without introducing bubbles. Using a wide-bore micropipette tip, 12 μL of 1:10 dilution was placed in each of the two ends of a hemocytometer. Four squares were counted on each side of the hemocytometer using a light microscope. Final cell counts were calculated using the following formula: average of the 8 squares x dilution factor x 104 x total volume = total number of protoplasts per milliliter of solution.
Microscope Images
10x and 40x bright field images were taken on an inverted light/fluorescence microscope pre and post sort to confirm protoplast intactness and enrichment of classes.
Cell size and distribution: Determine the expected size
range and distribution of the protoplasts before attempting to sort. Determining the distribution of protoplast sizes can assist in optimizing pre-sort procedures. In general, plant protoplasts can range from 10-150 μm in size depending on crop and tissue source. If the protoplast population is pushing the upper limit of the sortable size range for WOLF (see normal and left-skewed population examples in Figure 1 below), there are two options: (1) re-filter protoplasts through a specified filter size (e.g., 30- 40 μm) to eliminate the largest cell sizes or (2) increase the osmolarity of the sorting media to contract the cells.
Sorting Protoplasts with the WOLF
Protoplasts were diluted to a concentration of 2.5 x 105 cells per milliliter and kept in 100 x 25 mm petri dishes. When ready to sort, 3.0 mL of protoplasts were transferred to a sterile 5.0 mL FACS tube. The WOLF was primed and calibrated with sterile-filtered, distilled water and then re-primed with sterilefiltered protoplast MS final medium prior to analyzing and sorting protoplasts. PBS was not used as sheath buffer to sort protoplasts because the osmolarity is too low and leads to cell lysis. Sorting parameters are summarized in Table 1.
Plant protoplast classes were identified using chlorophyll autofluorescence and cell size estimated from scatter (Figure 4). These were then successfully separated via gentle sorting (Figure 5). All events shown above the 103 decade
are considered positive for chlorophyll and therefore likely plant cells and organelles. The parent gate encompasses chlorophyll-positive events (top left), and the child plot (top right, gated to chlorophyll positive events) shows the three independent gates for each of the three chlorophyll containing populations that were used for cell enrichment.
NOTE: It is suggested to pause the sort and invert the FACS tube gently every 15 minutes for the duration of the sort to keep protoplasts in suspension and to prevent cartridge clogging. Plant mesophyll protoplasts are denser than the sample buffer and sink rather quickly.
Results
The successfully sorted populations are shown by microscopy in Figure 5. Size ranges of the high- and low-chlorophyll protoplast populations are the same: 30-50 μm; while the cellfree chloroplasts are substantially smaller ~10 μm.
When analyzing the pre-sort sample, free chloroplasts are visible, which is an indication of cell lysis (Figure 4). However, after sorting, the sample contains undamaged protoplasts (Figure 5B and C). The low pressures in the fluidics (< 2 psi) paired with the use of protoplast culture medium as sheath provides an environment for a successful sort.
Conclusion
Enriching plant protoplasts can be challenging due to their variation in size, fragility, and sensitivity to shear stress and osmotic changes. A successful sort requires optimizing pre- and post-sort cell culture medium to support protoplast populations throughout the workflow (Figures 2 and 3). Results from these experiments demonstrate that the WOLF Cell Sorter can enrich plant protoplasts up to 50 μm in diameter and keep them intact and viable post sort (Figure 5). In addition, the WOLF was able to successfully identify and separate protoplast classes with varying chlorophyll levels, which is key for enriching different cell types that exist in plant leaves.
For more information, visit nanocellect.com or email [email protected]
References
1. Yoo, SD., Cho, YH. & Sheen, J. (2007). Arabidopsis mesophyll protoplasts: a versatile cell system for transient gene expression analysis. Nat Protoc 2,
1565–1572. doi:10.1038/nprot.2007.199
2. Shahin EA (1985). Totipotency of tomato protoplasts. Theor Appl Genet: 69(3):235-40. doi: 10.1007/BF00662431
3. Shepard JF, Totten RE (1975). Isolation and Regeneration of Tobacco Mesophyll Cell Protoplasts under Low Osmotic Conditions. Plant Physiol. 55(4):689-
94. doi:10.1104/pp.55.4.689
4. Reed KM, Bargmann BOR (2021). Protoplast Regeneration and Its Use in New Plant Breeding Technologies. Front Genome Ed. 3(734951):1-26.
doi:10.3389/fgeed.2021.734951
5. Ramahaleo T, Morillon R, Alexandre J, Lassalles JP (1999). Osmotic water permeability of isolated protoplasts. Modifications during development. Plant
Physiol. 119(3):885-96. doi: 10.1104/pp.119.3.885
APN-035